How Does Quantum Computing Differ from Classical Computing?
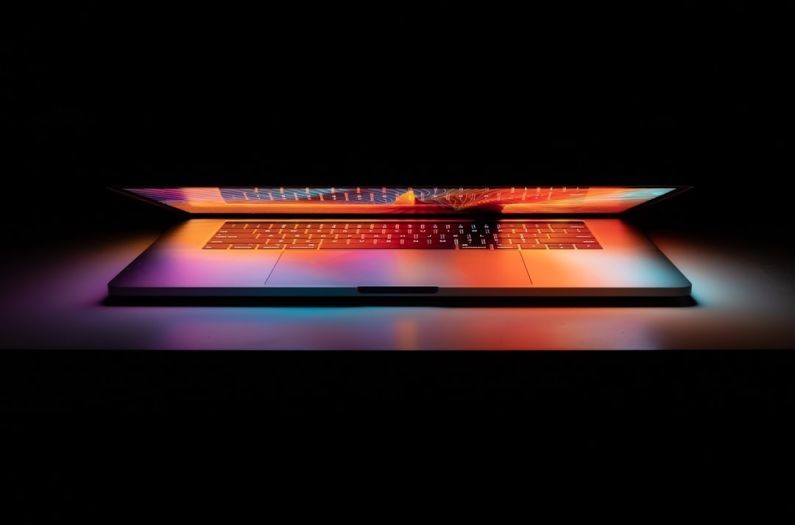
Quantum computing, a cutting-edge field at the intersection of quantum mechanics and computer science, has been gaining significant attention in recent years for its potential to revolutionize the way we process information. In contrast to classical computing, which relies on bits as the fundamental units of data storage and processing, quantum computing leverages quantum bits, or qubits, to perform computations in ways that were previously thought to be impossible. This article delves into the key differences between quantum computing and classical computing, shedding light on the unique principles that underpin each approach.
**Quantum Superposition vs. Classical Bits**
One of the most fundamental distinctions between quantum computing and classical computing lies in the way information is processed and stored. In classical computing, information is represented in bits, which can exist in one of two states: 0 or 1. These binary states form the basis of all classical computations, with each bit encoding a single piece of information. In contrast, quantum computing operates on qubits, which can exist in a state of superposition, meaning they can represent both 0 and 1 simultaneously.
This property of superposition allows quantum computers to perform multiple calculations at once, exponentially increasing their computational power compared to classical computers. While classical computers must process information sequentially, quantum computers can explore a multitude of possibilities in parallel, enabling them to solve complex problems much more efficiently.
**Entanglement and Quantum Parallelism**
Another defining feature of quantum computing is entanglement, a phenomenon in which the state of one qubit becomes intrinsically linked to the state of another, regardless of the physical distance between them. This interconnectedness enables quantum computers to exhibit a form of parallelism known as quantum parallelism, where operations on entangled qubits can influence each other instantaneously, leading to faster and more efficient computations.
In classical computing, parallelism is achieved through the use of multiple processors or cores working in tandem to execute tasks simultaneously. While this approach can enhance computational speed to a certain extent, it pales in comparison to the inherent parallelism offered by quantum entanglement. The ability of qubits to be entangled and interact with each other non-locally is a key advantage of quantum computing over classical computing.
**Quantum Algorithms and Speed**
Quantum computing also distinguishes itself through the use of quantum algorithms, which are specifically designed to leverage the unique properties of qubits to solve problems that are intractable for classical computers. One of the most famous quantum algorithms is Shor’s algorithm, which efficiently factors large numbers—a task that would take classical computers an impractical amount of time.
In addition to Shor’s algorithm, Grover’s algorithm is another notable quantum algorithm that can search unsorted databases in a time complexity of O(√n), providing a quadratic speedup compared to the linear time complexity of classical search algorithms. These quantum algorithms showcase the remarkable speed and efficiency gains that quantum computing can offer for certain types of problems.
**Challenges and Future Prospects**
Despite the enormous potential of quantum computing, there are significant challenges that must be overcome before it can become a mainstream technology. Quantum decoherence, which refers to the loss of quantum information due to interactions with the environment, remains a major hurdle in the development of practical quantum computers. Researchers are actively working on error correction techniques and quantum error correction codes to mitigate the effects of decoherence and improve the reliability of quantum computations.
Looking ahead, the future of quantum computing holds immense promise for a wide range of applications, from cryptography and optimization to drug discovery and materials science. As quantum technologies continue to advance and mature, we can expect to see a paradigm shift in computing capabilities, unlocking new possibilities and reshaping the way we approach complex problems in the digital age.